Objectives
Scientific Vision: Provide a validated methodology for estimating atmospheric loss from an arbitrary rocky planet that will serve the heliophysics, planetary, and exoplanetary communities.
There are many different kinds of planets, and many different kinds of stars. Which planets keep their atmospheres? MACH is devising a strategy to estimate how quickly different planets lose their atmosphere. To achieve our vision, the MACH Center has six science objectives, depicted in the figure.
- Improve and link models for atmospheric escape from any planet. Models necessary to compute rates from five atmospheric escape processes will be linked together, adding physical processes where necessary based on lessons learned from observations and theory.
- Use observations to validate models and probe escape physics. Observations of atmospheric escape from solar system planets will be used to validate the models, and will be comparatively analyzed to reveal new information about the processes that control escape.
- Compute stellar inputs for exoplanets and model transit light curves. We will derive the stellar driving conditions of escape for exoplanet-hosting stars, which will be used as input to our models for escape. The output of these models will then be used to simulate exoplanet transit light curves that can be compared with observations.
- Build a multi-dimensional model library for atmospheric escape. Our validated models for escape will be run over a large range of planetary and stellar parameters, building a multi-dimensional model library for both solar system objects and exoplanets.
- Develop an interactive web interface for estimating escape rates. We will develop a web interface tool to access MACH results, where any user can select planetary and stellar parameters and receive escape rate estimates based on the model library of all five loss processes, as well as access to the model results and data used to obtain the escape rates.
- Synthesize new understanding of atmospheric escape and its drivers. MACH’s goal is to move the helio community forward in understanding how atmospheric escape operates as a planetary process. We will synthesize new understanding in a book on escape.
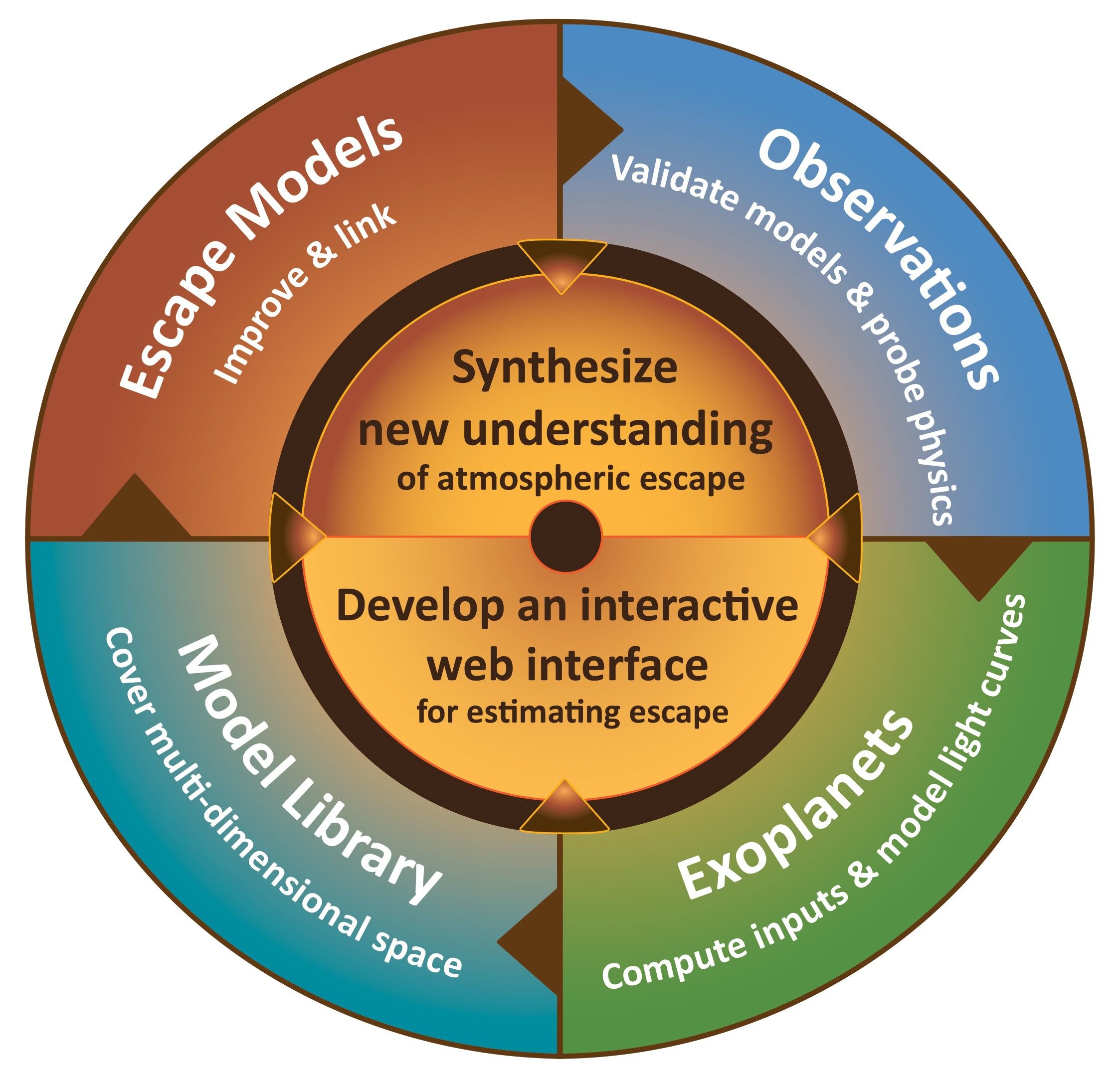
Escape Processes
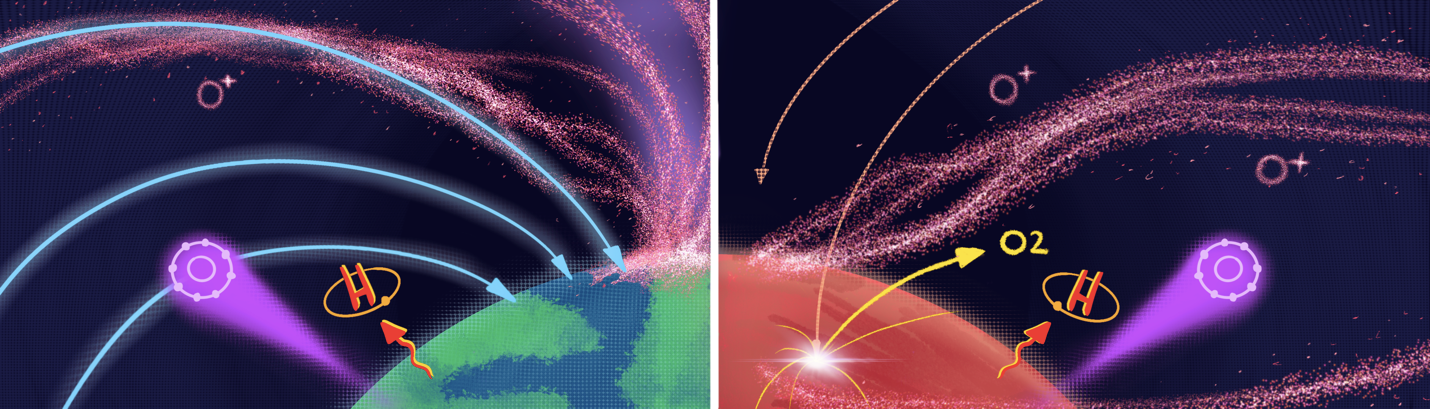
‘Atmospheric escape’ happens when atmospheric particles (atoms and molecules) have enough energy to escape the gravitational pull of a planet. This can happen in several different ways:
- Ion loss: Charged particles are accelerated by electric fields near the planet. Their motion is governed by both electric and magnetic fields.
- Thermal escape: In any collection of colliding gas particles, some have ‘above average’ energy and some have ‘below average’ energy. If the gas is hot enough, then some of the particles with ‘above average’ energy can escape.
- Hydrodynamic escape: An extreme version of thermal escape occurs when the atmosphere is very hot. When this occurs then atmospheric particles can ‘flow’ away to space in large quantities.
- Photochemical escape: Sunlight can drive chemical reactions in atmospheres. These reactions can break molecules apart, sending atoms into space.
- Sputtering: Energetic particles crashing into an atmosphere ‘splash’ atmospheric particles into space.
Atmospheric Escape from Exoplanets
Nearly 5000 exoplanets have been discovered in our galaxy, and we now realize that our galaxy hosts
hundreds of billions of planets. Which of them can retain atmospheres, increasing the chance that they
are habitable at their surface?
MACH includes both specific and hypothetical exoplanets in its work.
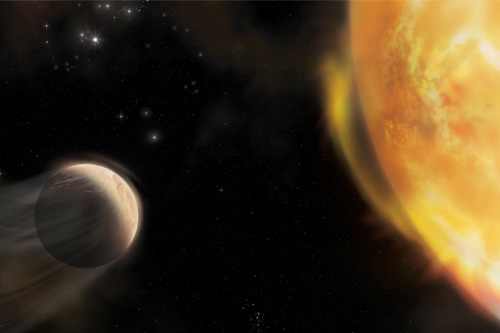
Exoplanet inputs
- Stellar spectra – We use observations of exoplanet-hosting stars to provide information about the amount of ultraviolet light coming from each star. UV light is important for driving atmospheric escape.
- Stellar winds – We use observations of exoplanet-hosting stars to drive models of the winds of charged particles flowing away from the star, as well as the magnetic field carried by the wind.
Predicting exoplanet observations
- Transit light curves – To estimate atmospheric escape from planets, MACH is using 3D models of the paths that particles take as they escape. The MACH team is using these model results to predict what telescope might observe as the planet (and its escaping atmosphere) passes in front of its star. This work can guide future observations and their interpretation.
MACH Method
The MACH team has devised a method for estimating atmospheric escape from any planet, illustrated in the figure.
Method
- Inputs for the atmosphere, planet, and star are collected. Inputs can be based on observations, or imposed arbitrarily.
- Upper atmosphere regions are modeled, based on the inputs. The main regions relevant for escape are the thermosphere, ionosphere, and exosphere.
- Energy transfer processes are incorporated into models and model results for both upper atmospheric regions and escape processes.
- Atmospheric escape processes are modeled, yielding estimates of atmospheric escape rates via each process.
- Observations and models are used to validate and verify each step along the way.
Strengths of this approach
- All escape processes are considered. Many studies today include only a subset of processes
- Multiple models are used wherever possible. This provides confidence in our estimates, and a sense of the uncertainties
- The approach is flexible. New models and model improvements can easily be accommodated.
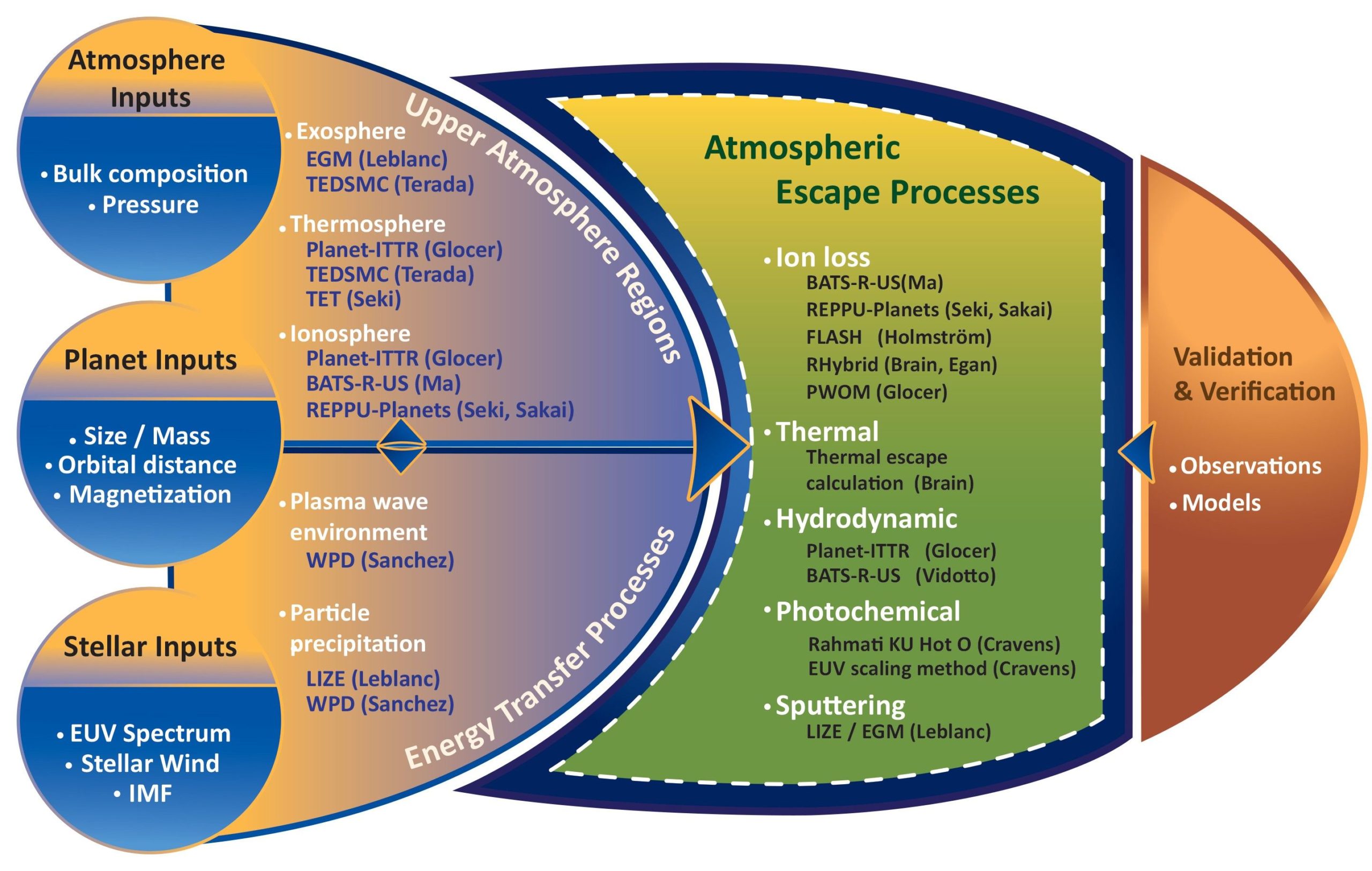
Models
Model Library
The MACH team will share its estimates of atmospheric escape with the large community. We are constructing a library of results for atmospheric escape that spans ranges of characteristics for the planet, its atmosphere, and its host star.
Star/Planet Characteristics | Range |
Stellar EUV spectrum | Active & inactive F,G,K,M stars |
Stellar wind | “low” / “moderate” / “high” |
Interplanetary magnetic field | Multi-species MHD |
Planet size and mass | ~Mercury/Titan -> “Super-Earth” |
Planet orbital distance | Habitable Zone distance/”Same stellar flux” |
Planet magnetization | 5 choices from “no dipole” to “> Earth dipole” |
Bulk atmosphere composition | CO2, H2 or N2 |
Surface pressure | Mercury -> Venus-like |
Interactive Web Tool
We are constructing an interactive web tool so that anyone can access MACH results. A user can select properties of the planet and star, and the tool will provide estimates of atmospheric escape based on MACH models.
Observation Data
The MACH Center’s work makes use of primary data from a number of scientific missions, as well as data and analysis from previously accomplished studies. Observations are being used both to better understand how atmospheric escape operates and to validate MACH estimates of atmospheric escape. For information on the missions that have been used to look into magnetic fields, ion escape and planetary atmosphere see their respective websites.